About
The Atom Interferometric Observatory and Network (AION) project is a multidisciplinary initiative to enable the exploration of ultra-light dark matter; searches for new fundamental interactions; and detection of gravitational waves from the early Universe and astrophysical sources in the previously unexplored mid-frequency band ranging from several mHz to a few Hz. The AION project will develop the technology to construct, operate and exploit new-generation atom interferometers. From the outset, a partnership with the Matter-wave Atomic Gradiometer and Interferometric Sensor (MAGIS) experiment at Fermi National Laboratory in the US has been established, thereby realising a detector network that will provide enhanced science opportunities not accessible to single detectors. The extremely high sensitivity of the matter-wave quantum sensors in AION arises from the superposition and interference of atomic wave packets and takes advantage of special features of strontium atoms used by state-of-the-art atomic clocks, in combination with established techniques for inertial sensors.
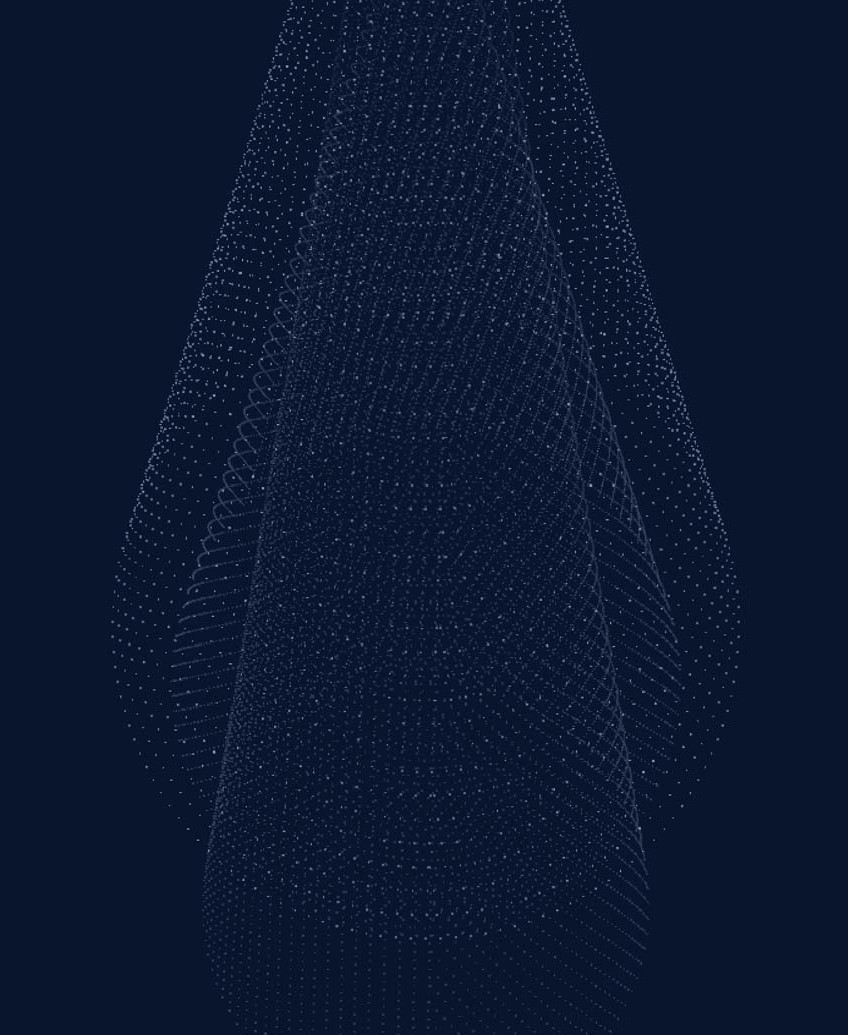

The long-term vision of the AION programme consists of 4 stages:
- Stage 1 is to build and commission a 10 m detector, develop existing technology and the infrastructure for the 100 m detector, including detailed plans and assessment of its performance.
- Stage 2 is to build, commission and exploit the 100 m detector and carry out a design study for the km-scale detector.
- Stage 3 will be a full-scale terrestrial (kilometre-scale) with a target of reaching the ultimate terrestrial sensitivity for dark matter and gravitational wave observation.
- Stage 4 looks towards a satellite-based (thousands of kilometres scale), detectors as the ultimate objective (for example the AEDGE mission).
The AION project is one of the key science initiatives supported by the UKRI/STFC/EPSRC Quantum Technologies for Fundamental Physics (QTFP) programme. Stage 1 of the project started in February 2021.
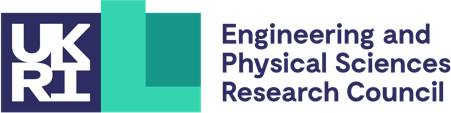
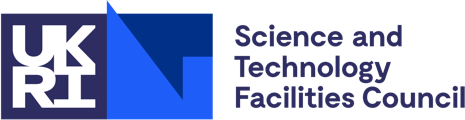
For more information:
- AION Collaboration, AION: An Atom Interferometer Observatory and Network, JCAP 05 (2020) 011, arXiv:1911.1175.
- MAGIS collaboration, Matter-wave Atomic Gradiometer Interferometric Sensor (MAGIS-100), arXiv:2104.02835
- Y.Abou El-Neaj et al., AEDGE: Atomic Experiment for Dark Matter and Gravity Exploration in Space, EPJ Quant. Tech 7 6 (2020).
- STFC Quantum Technologies for Fundamental Physics
- Ultra-cold Atom Technology
- AION-10 Pathfinder
- The MAGIS experiment
- Atom Interferometry in Space
- Physics
- National Quantum Technology Programme
Ultra-cold Atom Technology
Transport, Cooling and Atom Optics
AION is developing the technology to cool and transport large clouds of ultracold Strontium atoms with high efficiency and fast repetition rate, prepare the clouds for interferometer launch, and perform readout and analysis of the interferometer signal.
Interferometry sequences take place within a long, magnetically shielded vertical tube with a separation that defines the baseline of the differential measurement. Each cold atom cloud is prepared in a series of chambers collectively known as the sidearm, which are connected to the interferometry tube at critical intervals and host two stages of laser cooling in a magneto-optical trap. This is followed by evaporative cooling in an optical dipole trap formed at the intersection of two laser beams. The atoms are then trapped in the focus of a single laser beam and transported over 50 cm to the main interferometry chamber by translating the beam focus. A final stage of cooling in the interferometry chamber precedes the launch of the interferometry sequence, after which the phase information encoded in the recombined atom cloud is extracted by optimised imaging sequences and subsequent analysis.
Optimising the detector sensitivity requires atoms cooled to the lowest possible temperatures and with the fastest possible repetition rates. Our research will redefine the boundaries of this technology to enable ever more sensitive interferometer sequences.
Ultra-high Resolution Atom Interferometry
The AION detector measures the quantum phase between two arms of an atom interferometer. In our Ultra-High-Resolution Atom Interferometry work, we aim to measure this quantum phase with an unprecedented precision. Deploying high-phase-resolution techniques in a large-scale AION detector, we eventually hope to resolve tiny phase perturbations from passing dark matter or gravitational waves.
The phase measurement resolution in the atom interferometer is fundamentally limited by quantum projection noise. Upon measurement, each atom is projected into either the upper or the lower arm of the interferometer, and further information about the atom phase prior to measurement is lost. Assuming the atom interferometer contains N atoms in an unentangled state, the Standard Quantum Limit (SQL) places a lower limit δϕ=1/√N for the phase resolution. Therefore, one approach to Ultra-High-Resolution Atom Interferometry is to increase the number of atoms to the maximum amount possible - limited ultimately by the laser cooling sequence and atom-atom interactions. Performing this feat at a high repetition rate requires advances in the technology used to laser cool atoms, and so the AION collaboration will develop new high-flux sources of ultracold strontium to enable this work.
In parallel to increasing the atom number, we are also investigating techniques to entangle the atoms into useful "squeezed" states, which possess phase uncertainty below the SQL. The entanglement is generated by surrounding the atoms with a high-finesse cavity, and allowing the atoms to interact with each other through the photon field bouncing back and forth inside the cavity. Building on our earlier work on quantum non-demolition measurement in an atomic clock, we plan to squeeze the atomic state at least 20 dB below the SQL, and to launch the squeezed atoms into a proof-of-principle atom interferometer sequence.
High-fidelity Large Momentum Transfer
The AION consortium also focuses on the techniques and technology required to enable significant sensitivity improvements through implementing large momentum transfer (LMT). This allows the trajectories of the atom interferometry paths to separate more rapidly over time, allowing them to enclose a greater space-time area – making them better able to detect signals of interest such as dark matter or gravitational waves.
The atom interferometer is created using a series of pulses to impart momentum to the atoms. After first placing the atom into a quantum superposition, such that it has a wave function moving along two trajectories, a series of pulses will be applied. The roles of light and matter are inverted, such that the atoms now play the role of a beam of light in a conventional optical interferometer. Each pulse can then be thought of as a mirror with a certain reflectance – or fidelity. If the mirror pulse is not perfectly reflective, this introduces losses that result in decoherence. To achieve the goals of the first stage of AION, we are working on how to make these pulses better than 99.9% efficient, and aim to achieve momentum transfers of the order 1000 ħk, with ħk being the amount of recoil momentum transferred by a photon.
To achieve this, we are investigating novel pulse schemes and time-domain approaches to reduce decoherence and improve fidelity, as well as active, closed loop, wave front control focusing on addressing middle-spatial frequency aberrations. Furthermore, we are investigating approaches to optimise beam delivery, including beam shaping and enhancement, and realisation of improved optical intensities to enable the use of shorter mirror pulses, including for future clock transition operation. Furthermore, we are developing schemes for atom interferometry to address challenges such as how to open and close the atom interferometer, and to limit effects such as spontaneous emission or increase resilience over long base lines. In addition to experimental demonstrations of LMT, we are developing models for the atom interferometer fidelity and performance budget, and using these to design the requirements of the optical and atomic systems for future stages of AION.
AION-10 Pathfinder
The AION-10 pathfinder device will be the first large-scale atom-interferometer experiment in the UK. This instrument will consist of two atom interferometers, located one above the other, and housed within a 10 m tall vacuum system. Atom sources at the bottom and middle of the device will prepare the laser-cooled atom clouds required for the interferometry sequence. To make a measurement, these clouds are launched upwards. Laser pulses running through the device will both split and recombine atom wave packets from these clouds to create each interferometer. By using the same laser pulses to address both atom interferometers and taking the difference in signal between them, many technical noise sources can be eradicated to greatly improve the sensitivity of the device. The AION-10 device will allow us to explore new regions of dark matter parameter space, and also demonstrate the technologies and integration required to build even larger detectors in the future.
The chosen site for the AION-10 pathfinder is situated in the newly-constructed low-vibration Beecroft building in Oxford. The site provides world-class infrastructure, including a 15m shaft and an adjacent high-specification laser lab that will be used to produce the required laser light for this project.
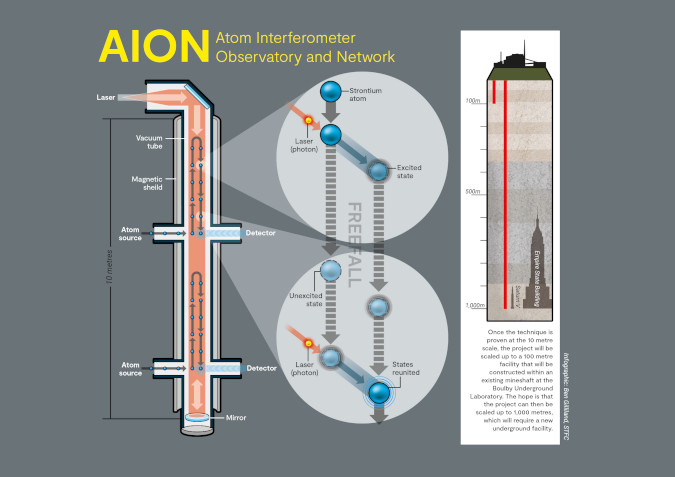
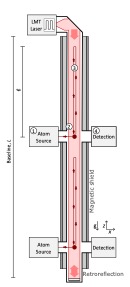
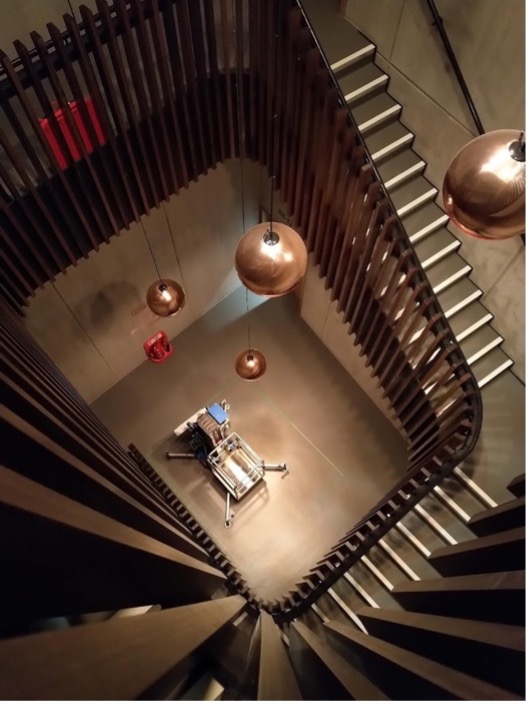
MAGIS-100 Experiment
MAGIS-100 is a next-generation quantum sensor under construction at Fermilab that aims to explore fundamental physics with atom interferometry over a 100-meter baseline. This novel detector will search for ultralight dark matter, test quantum mechanics in new regimes, and serve as a technology pathfinder for future gravitational wave detectors in a previously unexplored frequency band. It combines techniques demonstrated in state-of-the-art 10-meter-scale atom interferometers with the latest technological advances of the world's best atomic clocks. MAGIS-100 will provide a development platform for a future kilometer-scale detector that would be sufficiently sensitive to detect gravitational waves from known sources. The UK will deliver the detection system for the MAGIS interferometer and its associated readout, this will also be integrated into the AION experiment.

Atom Interferometry in Space
AEDGE (Atomic Experiment for Dark Matter and Gravity Exploration) is a concept for a space mission using the same principles of atom interferometry as AION to explore further the nature of dark matter and measure gravitational waves with frequencies between 1 and 0.01 Hz, where existing and planned experiments lose sensitivity. Consisting of two satellites in medium earth orbit about 40,000 km apart, AEDGE would probe the couplings to electrons and photons of ultralight scalar dark matter particles weighing between 10-12 and 10-19 electron-Volts, with a sensitivity reaching some 10 orders of magnitude greater than current limits. The searches for gravitational waves with AEDGE would be sensitive to the mergers of intermediate-mass black holes and could cast light on the way in which the supermassive black holes inside many galaxies could have been formed. AEDGE could also search for a stochastic background of gravitational waves, as might have been generated by a first-order transition in the early Universe, or cosmic strings. The AEDGE concept has been discussed in international workshops (Workshop July 2019, Workshop Sep 2021) and was presented to the European Space Agency in response to its Voyage 2050 call for new mission concepts.
For more information:
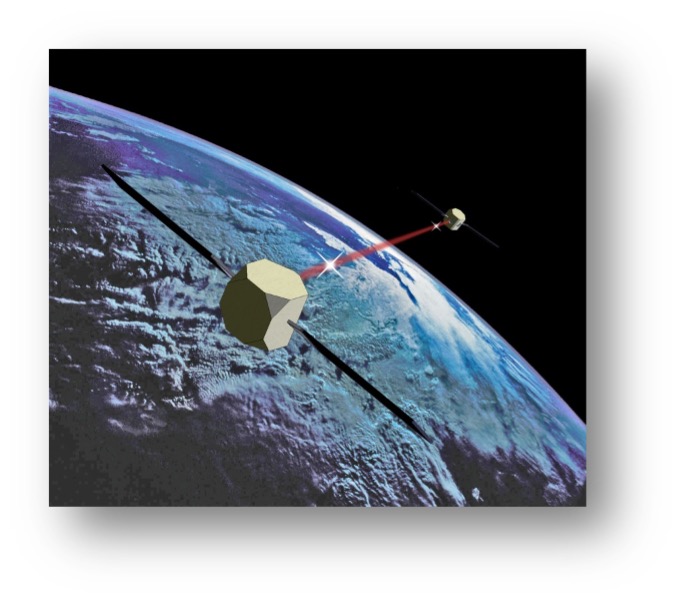
AION Physics
It is expected that ultra-light dark matter can lead to a measurable change in the phase differences between quantum superpositions of spatially separated clouds of strontium atoms. The physics work package team is undertaking studies to guide the design of AION-10. We are working to understand the effects of changing experimental parameters on the sensitivity to ultra-light dark matter. We anticipate that after a few years of data-taking, AION-10 will test how ultra-light dark matter that is around 100-billion-billion times lighter than the electron mass interacts with electrons in the strontium atom. In addition to these design studies, the physics work package team is also undertaking studies to investigate the degree to which the characteristic signatures of ultra-light dark matter are imprinted onto the measured signals in AION-10. These characteristic signals arise from the motion of the Earth through the dark matter in our galaxy. These studies are needed to disentangle potential dark matter and gravitational wave signals in the long-baseline interferometers that will come after AION-10.
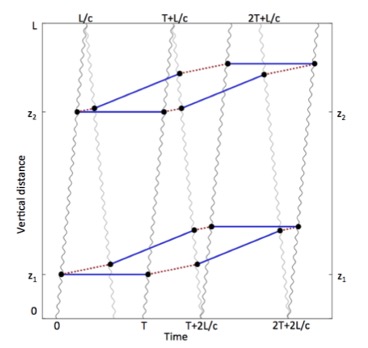
National Quantum Technology Hub
The UK Quantum Technology Hub Sensors and Timing (led by the University of Birmingham) brings together world-leading experts from physics and engineering from the Universities of Birmingham, Glasgow, Imperial College London, Liverpool John Moores, Nottingham, Southampton, Strathclyde and Sussex, NPL and the British Geological Survey to drive commercial exploitation of quantum sensor technology in collaboration with industry. Particular areas of focus are magnetometry, geophysics, navigation, timing and underpinning technology aimed at reducing the size, weight power and cost of future sensor systems.
Set up in 2014, the QT Hub Sensors and Timing ultimately aims to unlock a new range of sensing capabilities, urgently needed to strengthen the services which form our critical infrastructure – construction, transport, communications, and other key sectors - with quantum sensors and timing devices. Academics across the consortium are developing small, portable and cost-effective quantum sensor technology to enable resilient and robust innovative technology for the future.
The QT Hub, which has over 100 projects valued at approximately £100 million, is keen to collaborate with industry companies to further advance quantum sensor technologies.
The UK Quantum Technology Hub Sensors and Timing is part of the National Quantum Technologies Programme (NQTP), which was established in 2014 and has EPSRC, IUK, STFC, MOD, NPL, BEIS, and GCHQ as partners.
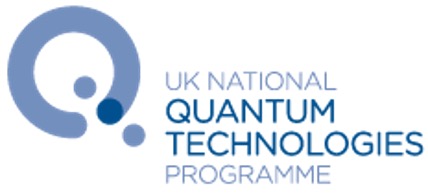
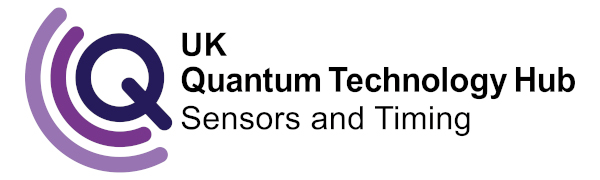
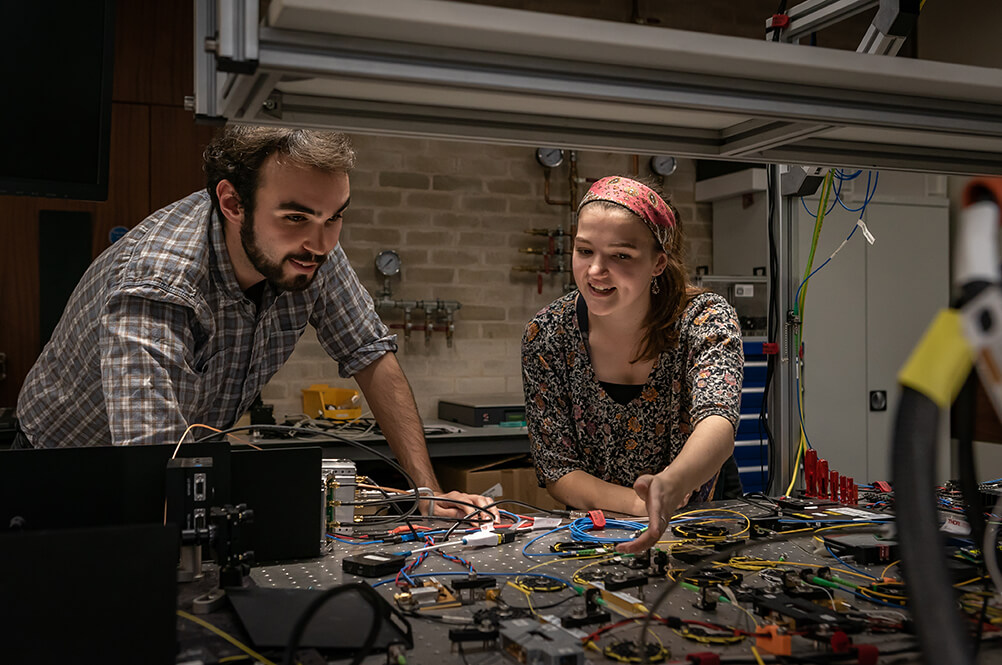
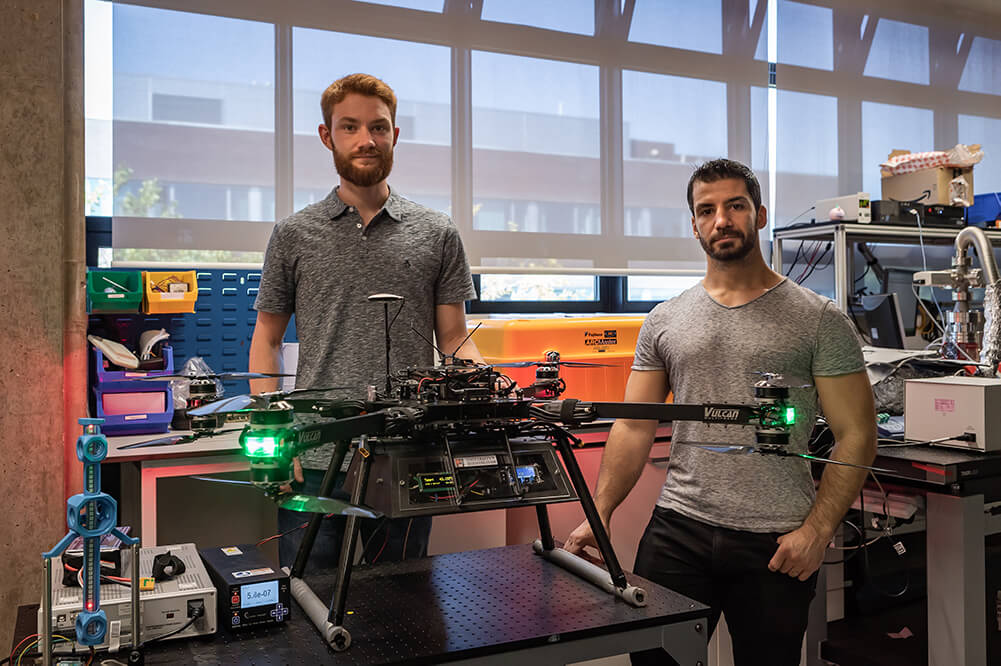